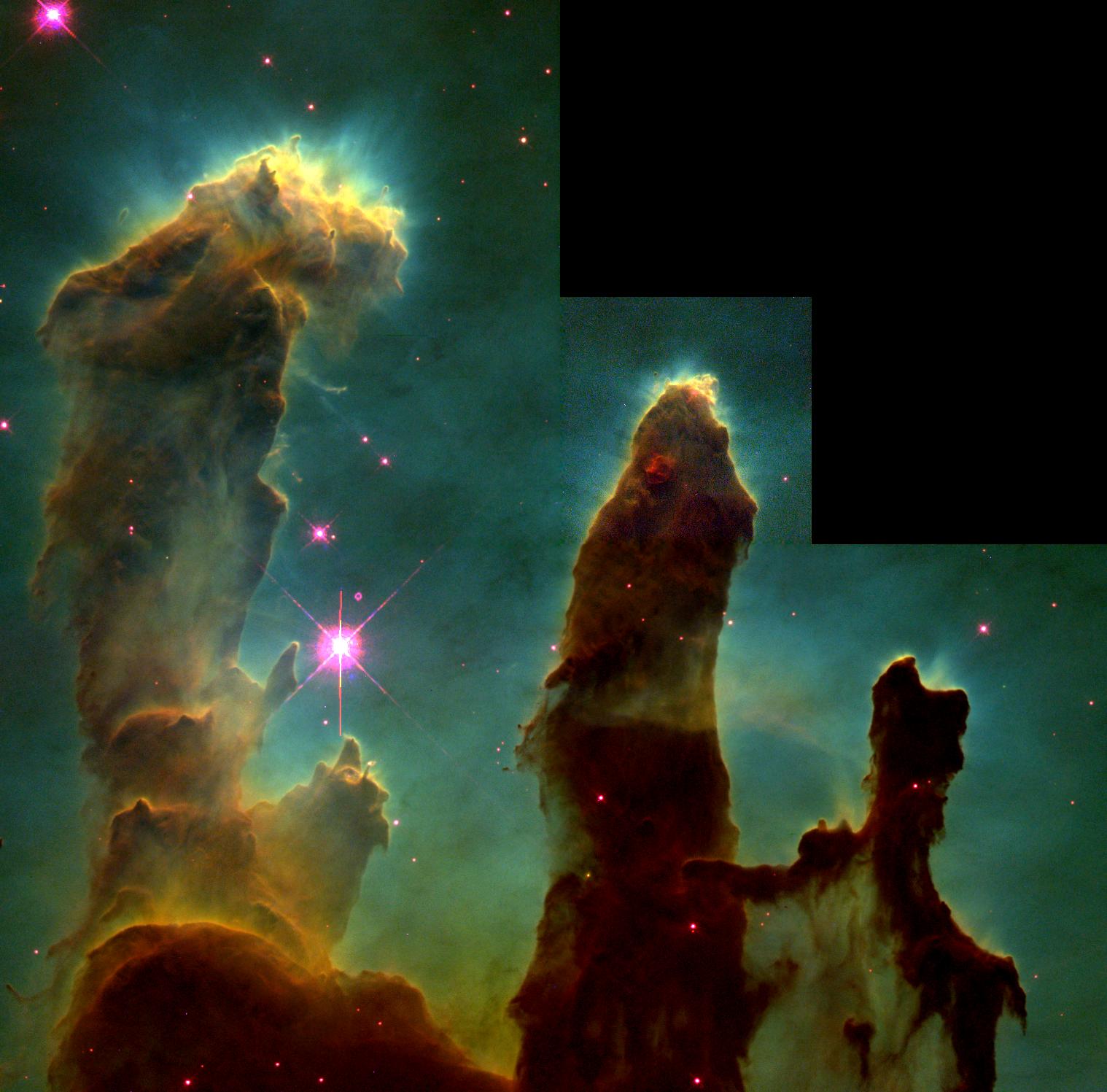
The “Special Topics” presentation two weeks ago pointed out that the term “small bodies” of the solar system generally refers to objects like comets and asteroids, although that particular presentation discussed another class of such objects, i.e., the small moons of the planets. To carry the term “small bodies” to an extreme, we could examine what are certainly the “smallest bodies” of all, i.e., the individual grains of dust that are within the solar system, the majority of which are in orbit around the sun like most of the other objects. It turns out that these dust grains are inextricably linked with the more conventional “small bodies” of the solar system, and thus it is entirely appropriate to include some discussion about them.
Dust has been within our solar system since the very beginning. Indeed, it was out of large interstellar clouds of dust and gas that the solar system formed in the first place, and we see numerous examples of these structures all over the sky, especially within the plane of our Galaxy (which appears as the hazy band of light that we call the “Milky Way,” which is made up of the combined light of multitudes of distant stars in the galactic plane). Much of that dust was used in forming the sun and the planets, much of it by way of the smaller objects, i.e., the planetesimals that combined to build the planets; the “leftover” such objects survive today as comets and asteroids. While a fair amount of that original dust would also have been left over, various processes including solar radiation pressure and “sweeping up” by the planets, as well as the solar system’s overall travels through interstellar space, would have removed the vast majority of it during the solar system’s lifetime. Meanwhile, since dust pervades interstellar space the solar system is constantly passing through it in the course of its travels. Such interstellar dust can be detected via its compositional and isotopic structure, and a few of the dust samples returned to Earth by the Stardust mission – which had flown through the coma of Comet 81P/Wild 2 in early 2004 and returned to Earth two years later – have been found to be interstellar in origin.
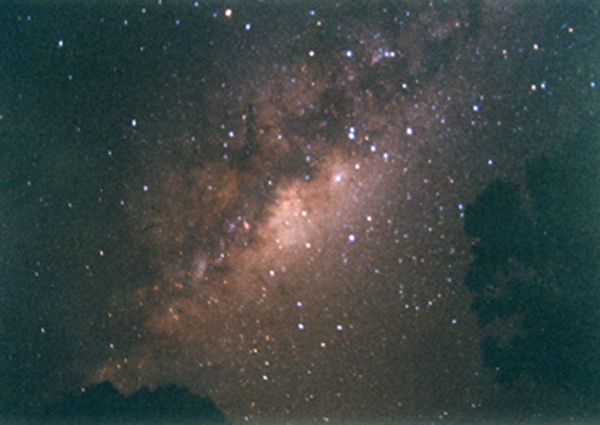
Since there is undoubtedly a lot of dust in our solar system today, there must accordingly be means of producing dust that remain operational. One of the largest sources of dust in the solar system is comets. As discussed in a previous “Special Topics” presentation on the structure of a comet’s nucleus, one of the biggest constituents of a nucleus is dust, usually embedded within the ices that are another one of the major constituents. As a comet approaches the sun and this ice begins to sublimate and erupt off the nucleus, the embedded dust grains are ejected as well. While some of this dust may eventually fall back upon the nucleus, the rest of it – which we may see as the comet’s dust tail – never returns, but instead follows along with the comet in the same basic orbit. In 1983 the InfraRed Astronomical Satellite (IRAS) spacecraft detected some of these dust trails accompanying various comets.
If Earth should encounter these streams of cometary dust during its annual orbit around the sun, we see this as a meteor shower. The relationship between comets and meteor showers is examined more thoroughly in a future “Special Topics” presentation.

Over the lifetime of the solar system many, many comets have visited the inner solar system and have become active – many comets doing so repeatedly – and over time these dust streams spread out. By now, the entire inner solar system is awash in cometary dust. The “sporadic” meteors, i.e., those meteors that do not appear to be associated with any known shower, that we see throughout the course of any given night are reminders of the presence of this dust.
Collisions and impacts between asteroids are another source of interplanetary dust. The various asteroids that have been examined by spacecraft all exhibit craters that attest to impact events, and over the fairly recent past we have witnessed the aftermath of apparent impacts. (These events are discussed more thoroughly in a future “Special Topics” presentation on “active asteroids.”) The existence of asteroid “families” – which will be discussed in another future “Special Topics” presentation – also attests to such impact events. Meanwhile, other “active asteroids” appear to be spewing dust as a result of a rapid rotation, which in turn is driven at least in part by the pressure of sunlight.

in actuality an “active asteroid” spewing streams of dust due to sunlight-induced rapid rotation. Courtesy NASA/ESA/David Jewitt.
As already mentioned, when Earth encounters these grains of dust we see them – at least, the larger ones – as meteors. The relative speeds are on the order of ten km per second, and the grains begin to disintegrate as a result of friction with the atmosphere. In truth, what we actually observe when we see a meteor are the molecules of the surrounding air that have been ionized by the heat generated by this friction. The dust grains themselves do not completely disintegrate but instead are broken down into even smaller particles that remain in the upper atmosphere, eventually raining down onto the surface – which anyone who has ever tried to keep any space clean and free of dust has encountered. The amount of dust that rains onto the surface has been measured by various means, all the way from radar observations of meteors to dust extracted from core samples of polar ice, with somewhat discordant results that range from 5 to as high as 100 to 200 tons per day. The resolution of these various measurements remains an area of active research.

The “noctilucent clouds” that are visible from higher latitudes during the respective hemispheres’ summer months are formed when water vapor brought up from the lower atmosphere condenses into ice crystals around these tiny dust grains in the mesosphere 80 km above the surface. Meanwhile, during a series of high-altitude aircraft flights equipped with special collectors during the early- to mid-1970s, University of Washington scientist Donald Brownlee was able to collect and subsequently examine numerous examples of these small grains. These “Brownlee particles,” as they were dubbed, were found to be, on average, a few microns in diameter, and to be puffy, porous aggregates of yet smaller particles with compositions similar to those of stony meteorites. The Brownlee particles were also found to be very dark, about as dark as soot, which has since been found to be consistent with the very dark surfaces of comet nuclei, which over time become coated with the ejected dust that manages to fall back onto the surface.
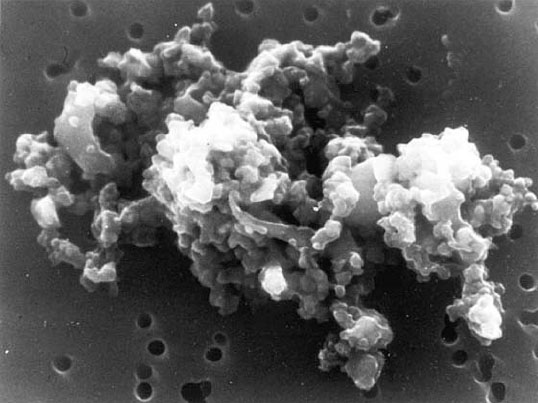
In space this dust presents a hazard to spacecraft. Even though these “micrometeoroids” are very tiny, the relative impact velocities are quite high – again, on the order of 10 km per second – and since kinetic energy goes as the square of the velocity, even tiny particles can cause damage. Orbiting scientific spacecraft, like the Hubble Space Telescope, need to be shielded from the effects of impacts by these objects, as do all space missions involving human crews.
Even though the individual particles that make up the population of interplanetary dust are very dark, they still reflect the sunlight that strikes them, and thus collectively they can be visible by that reflected sunlight. They can best be detected from Earth’s surface in the western sky after dusk and in the morning sky before dawn, as a vague, cone-shaped patch of light along the plane of Earth’s orbit, or ecliptic, dubbed the “zodiacal light.” From dark rural locations the zodiacal light can be seen on almost any clear moonless night, although the best occasions for viewing occur when the ecliptic makes a steep angle with respect to the horizon and when the zodiacal light is not superposed onto the Milky Way. From my personal experience, in the northern hemisphere (at a southern temperate latitude) the zodiacal light is most prominent in the evening sky during January and February and in the morning sky during September.
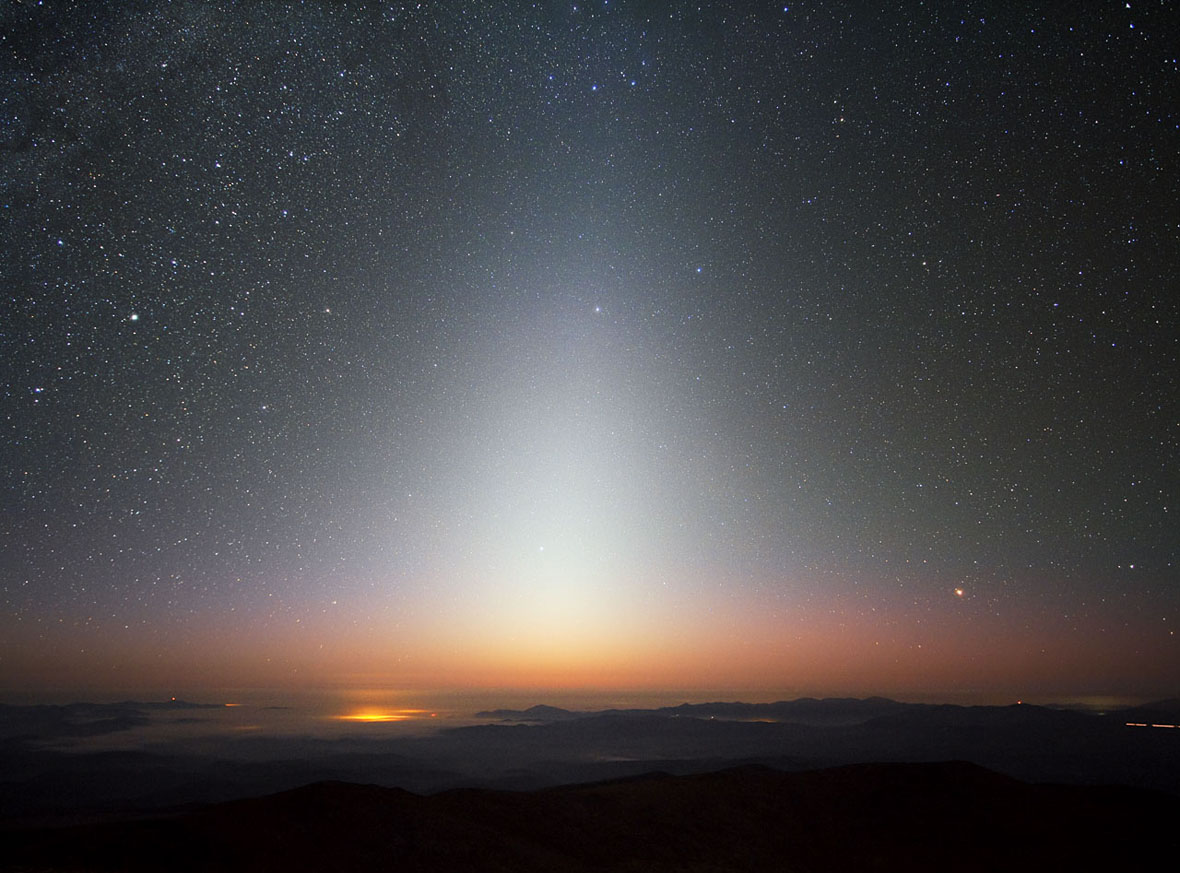
The dust that is located beyond Earth in the opposite direction from the sun can reflect sunlight back to Earth, and this is visible as a very vague and wide – on the order of ten degrees in diameter – patch of light called the “gegenschein” (from German words meaning “counter-glow”). As is the case with zodiacal light, in theory the gegenschein can be seen from dark rural sites on almost any given clear moonless night, although it is best visible when it is not superposed on the Milky Way and/or several bright stars. From my personal experience, the gegenschein is best visible around the time of the September equinox, when it is visible against a patch of relatively empty sky in Pisces south of the “Great Square” of Pegasus. On the clearest nights a very vague and weak band of light – the “zodiacal band” – can be seen connecting the zodiacal light with the gegenschein.
An even more elusive collection of interplanetary dust are the “Kordylewski clouds,” first reported by the Polish astronomer Kazimierz Kordylewski in 1961 and located near the Earth-moon L4 and L5 Lagrangian points. Their existence has remained controversial ever since Kordylewski’s initial reports, with many observers failing to detect them, however they appear to have been confirmed in 2018 by a team of Hungarian researchers led by Gabor Horvath of Eotvos Lorand University in Budapest. Horvath’s team made their observations with a linear polarizing filter attached to a sensitive CCD and detected an extended but very diffuse cloud located at L5.

All this interplanetary dust does not remain in one place, of course, but like everything else in the solar system it is constantly in motion. The various motions exhibited by this dust have been examined by, among others, British astronomer Brian May, who first began examining it during the early 1970s before taking a few decades off during which he was the lead guitarist for the rock band Queen, but he later returned to it to complete his doctoral dissertation in 2007. May found that, as expected, the dust primarily exhibits a direct orbital motion around the sun, however this exhibits an asymmetric pattern, and there are other discrete components that indicate collisions between asteroids and/or the effects of passing comets. There is also a likely component, the strength of which is unknown, that is due to interstellar dust that the solar system is currently passing through. In essence, as is true with many other facets of science the reality is more complex than what might be apparent at first glance – which in turn creates more opportunity for study and for future discoveries.

More from Week 35:
This Week in History Comet of the Week Free PDF Download Glossary
Ice and Stone 2020 Home Page